- Date
- 2025.02.27.
- Hits
- 64
Professor Moonsang Lee’s Research Team Presents World’s First Method to Maximize Plasmonic Hot Electron Photoconversion
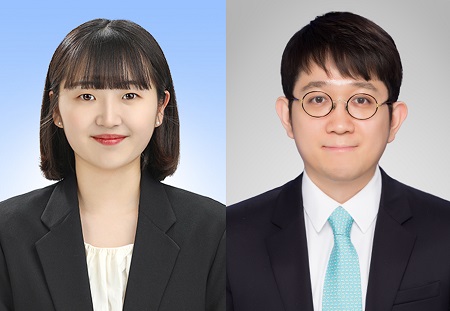
First Experimental Verification of Mechanism for Enhancing Photoconductivity Using Plasmonic Hot Electrons
Potential for Next-Generation Energy Conversion Devices Such as LiDAR and Image Sensors
▲ (From left: Jihyang Park, Department of Materials Science and Engineering, and Professor Moonsang Lee, Department of Materials Science and Engineering)
Professor Moonsang Lee’s research team from the Department of Materials Science and Engineering has recently identified—for the first time in the world—a physical mechanism that overcomes the limitations of conventional photoconductive properties using hot electrons.
Photodetectors, which convert light energy (solar energy) into electrical energy using semiconductor materials, are widely used in solar cells, LiDAR sensors, and image sensors. While these devices allow for the utilization of infinite solar energy, their performance improvements have been hindered by limitations in light absorption capacity and energy conversion efficiency.
Researchers have continuously sought ways to increase photoconversion efficiency, leading to growing interest in hot-electron-based photodetectors as a next-generation energy conversion concept.
Hot electrons are high-energy electrons generated when light energy irradiates a metal surface. These electrons can be used to convert solar energy into an electric current, unlike conventional photodetectors, which minimize energy loss. However, the extremely short lifespan of hot electrons—only a few picoseconds (1 trillionth of a second)—and their limited diffusion distance of just a few tens of nanometers make their collection and practical application challenging.
To harness hot electrons in photodetectors, the research team devised a strategy to minimize drawbacks and maximize advantages.
They proposed a method to capture hot charges generated by plasmon resonance within deep-level defects of a semiconductor, thereby enhancing photovoltaic effects and photoconductivity and maximizing energy conversion efficiency.
By fabricating a metal-semiconductor nano-diode composed of gold-based metal nanostructures and n-type gallium nitride, the team demonstrated their theory in practice.
Key Experimental Findings
Their study showed that hot electrons generated from localized surface plasmon resonance (LSPR) in the metal nanostructure were effectively captured within the deep defect levels of the semiconductor substrate. As a result, the photovoltaic effect and photoconductivity improved significantly, leading to an approximately two-fold increase in energy conversion efficiency compared to devices without hot charge capture.
This research is notable for systematically identifying the fundamental cause of hot-electron-induced photoconductive phenomena despite the experimental limitations associated with the ultrashort lifespan of plasmonic hot electrons.
Additionally, the study expanded the application potential of hot electrons beyond photocatalysis, demonstrating their direct applicability in optoelectronic devices.
The findings are expected to contribute significantly to the development of next-generation high-efficiency semiconductor devices, optoelectronic devices, and photocatalysts.
Professor Moonsang Lee stated:
"This research highlights the potential of hot electrons in optoelectronic applications. We believe it will serve as a foundational study for advancing next-generation energy conversion devices based on hot charge carriers."
This research was recently published as a cover paper in the globally renowned materials science journal, Nano Letters.
The study was co-authored by Jihyang Park, a student in the Department of Materials Science and Engineering, and Dr. Yujin Park from the University of Texas at Austin, as joint first authors. Professors Moonsang Lee (Inha University) and Eungyu Kim (Hanyang University) served as co-corresponding authors.
▲ Schematic representation of plasmonic hot electron behavior in metal nanostructures under varying light wavelengths. At a wavelength of 808 nm, surface plasmon resonance generates
an increased number of hot electrons, which interact with semiconductor defects, enhancing both charge capture and optoelectronic properties.
- Attached file
- There is no file attached.